Transcription factor
Transcription factor glossary |
---|
• gene expression – the process by which information from a gene is used in the synthesis of a functional gene product such as a protein |
• transcription – the process of making RNA from a DNA template by RNA polymerase |
* transcription factor – a protein that binds to DNA and regulates gene expression by promoting or suppressing transcription |
• transcriptional regulation – controlling the rate of gene transcription for example by helping or hindering RNA polymerase binding to DNA |
• upregulation, activation, or promotion – increase the rate of gene transcription |
• downregulation, repression, or suppression – decrease the rate of gene transcription |
• coactivator – a protein that works with transcription factors to increase the rate of gene transcription |
• corepressor – a protein that works with transcription factors to decrease the rate of gene transcription |
• response element – a specific sequence of DNA that a transcription factor binds to |
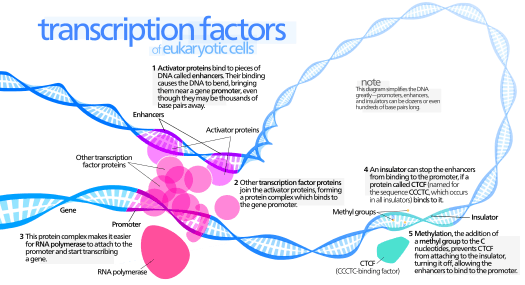
In molecular biology, a transcription factor (or sequence-specific DNA-binding factor) is a protein that controls the rate of transcription of genetic information from DNA to messenger RNA, by binding to a specific DNA sequence.[1][2] In turn, this helps to regulate the expression of genes near that sequence. This is essential in embryogenesis.
Transcription factors work alone or with other proteins in a complex, by promoting (as an activator), or blocking (as a repressor) the recruitment of RNA polymerase (the enzyme that performs the transcription of genetic information from DNA to RNA) to specific genes.[3][4][5]
A defining feature of transcription factors is that they contain at least one DNA-binding domain (DBD), which attaches to a specific sequence of DNA adjacent to the genes that they regulate.[6][7] Other proteins such as coactivators, chromatin remodelers, histone acetyltransferases, histone deacetylases, kinases, and methylases, while also essential to gene regulation, lack DNA-binding domains, and, therefore, are not transcription factors.[8]
Conservation in different organisms
Transcription factors are essential for the regulation of gene expression and are, as a consequence, found in all living organisms. The number of transcription factors found within an organism increases with genome size, and larger genomes tend to have more transcription factors per gene.[9]
There are approximately 2600 proteins in the human genome that contain DNA-binding domains, and most of these are presumed to function as transcription factors,[10] though other studies indicate it to be a smaller number.[11] Therefore, approximately 10% of genes in the genome code for transcription factors, which makes this family the single largest family of human proteins. Furthermore, genes are often flanked by several binding sites for distinct transcription factors, and efficient expression of each of these genes requires the cooperative action of several different transcription factors (see, for example, hepatocyte nuclear factors). Hence, the combinatorial use of a subset of the approximately 2000 human transcription factors easily accounts for the unique regulation of each gene in the human genome during development.[8]
Mechanism
Transcription factors bind to either enhancer or promoter regions of DNA adjacent to the genes that they regulate. Depending on the transcription factor, the transcription of the adjacent gene is either up- or down-regulated. Transcription factors use a variety of mechanisms for the regulation of gene expression.[12] These mechanisms include:
- stabilize or block the binding of RNA polymerase to DNA
- catalyze the acetylation or deacetylation of histone proteins. The transcription factor can either do this directly or recruit other proteins with this catalytic activity. Many transcription factors use one or the other of two opposing mechanisms to regulate transcription:[13]
- histone acetyltransferase (HAT) activity – acetylates histone proteins, which weakens the association of DNA with histones, which make the DNA more accessible to transcription, thereby up-regulating transcription
- histone deacetylase (HDAC) activity – deacetylates histone proteins, which strengthens the association of DNA with histones, which make the DNA less accessible to transcription, thereby down-regulating transcription
- recruit coactivator or corepressor proteins to the transcription factor DNA complex[14]
Function
Transcription factors are one of the groups of proteins that read and interpret the genetic "blueprint" in the DNA. They bind to the DNA and help initiate a program of increased or decreased gene transcription. As such, they are vital for many important cellular processes. Below are some of the important functions and biological roles transcription factors are involved in:
Basal transcription regulation
In eukaryotes, an important class of transcription factors called general transcription factors (GTFs) are necessary for transcription to occur.[15][16][17] Many of these GTFs do not actually bind DNA, but rather are part of the large transcription preinitiation complex that interacts with RNA polymerase directly. The most common GTFs are TFIIA, TFIIB, TFIID (see also TATA binding protein), TFIIE, TFIIF, and TFIIH.[18] The preinitiation complex binds to promoter regions of DNA upstream to the gene that they regulate.
Differential enhancement of transcription
Other transcription factors differentially regulate the expression of various genes by binding to enhancer regions of DNA adjacent to regulated genes. These transcription factors are critical to making sure that genes are expressed in the right cell at the right time and in the right amount, depending on the changing requirements of the organism.
Development
Many transcription factors in multicellular organisms are involved in development.[19] Responding to stimuli, these transcription factors turn on/off the transcription of the appropriate genes, which, in turn, allows for changes in cell morphology or activities needed for cell fate determination and cellular differentiation. The Hox transcription factor family, for example, is important for proper body pattern formation in organisms as diverse as fruit flies to humans.[20][21] Another example is the transcription factor encoded by the Sex-determining Region Y (SRY) gene, which plays a major role in determining sex in humans.[22]
Response to intercellular signals
Cells can communicate with each other by releasing molecules that produce signaling cascades within another receptive cell. If the signal requires upregulation or downregulation of genes in the recipient cell, often transcription factors will be downstream in the signaling cascade.[23] Estrogen signaling is an example of a fairly short signaling cascade that involves the estrogen receptor transcription factor: Estrogen is secreted by tissues such as the ovaries and placenta, crosses the cell membrane of the recipient cell, and is bound by the estrogen receptor in the cell's cytoplasm. The estrogen receptor then goes to the cell's nucleus and binds to its DNA-binding sites, changing the transcriptional regulation of the associated genes.[24]
Response to environment
Not only do transcription factors act downstream of signaling cascades related to biological stimuli but they can also be downstream of signaling cascades involved in environmental stimuli. Examples include heat shock factor (HSF), which upregulates genes necessary for survival at higher temperatures,[25] hypoxia inducible factor (HIF), which upregulates genes necessary for cell survival in low-oxygen environments,[26] and sterol regulatory element binding protein (SREBP), which helps maintain proper lipid levels in the cell.[27]
Cell cycle control
Many transcription factors, especially some that are proto-oncogenes or tumor suppressors, help regulate the cell cycle and as such determine how large a cell will get and when it can divide into two daughter cells.[28][29] One example is the Myc oncogene, which has important roles in cell growth and apoptosis.[30]
Pathogenesis
Transcription factors can also be used to alter gene expression in a host cell to promote pathogenesis. A well studied example of this are the transcription-activator like effectors (TAL effectors) secreted by Xanthomonas bacteria. When injected into plants, these proteins can enter the nucleus of the plant cell, bind plant promoter sequences, and activate transcription of plant genes that aid in bacterial infection.[31] TAL effectors contain a central repeat region in which there is a simple relationship between the identity of two critical residues in sequential repeats and sequential DNA bases in the TAL effector’s target site.[32][33] This property likely makes it easier for these proteins to evolve in order to better compete with the defense mechanisms of the host cell.[34]
Regulation
It is common in biology for important processes to have multiple layers of regulation and control. This is also true with transcription factors: Not only do transcription factors control the rates of transcription to regulate the amounts of gene products (RNA and protein) available to the cell but transcription factors themselves are regulated (often by other transcription factors). Below is a brief synopsis of some of the ways that the activity of transcription factors can be regulated:
Synthesis
Transcription factors (like all proteins) are transcribed from a gene on a chromosome into RNA, and then the RNA is translated into protein. Any of these steps can be regulated to affect the production (and thus activity) of a transcription factor. An implication of this is that transcription factors can regulate themselves. For example, in a negative feedback loop, the transcription factor acts as its own repressor: If the transcription factor protein binds the DNA of its own gene, it down-regulates the production of more of itself. This is one mechanism to maintain low levels of a transcription factor in a cell.
Nuclear localization
In eukaryotes, transcription factors (like most proteins) are transcribed in the nucleus but are then translated in the cell's cytoplasm. Many proteins that are active in the nucleus contain nuclear localization signals that direct them to the nucleus. But, for many transcription factors, this is a key point in their regulation.[35] Important classes of transcription factors such as some nuclear receptors must first bind a ligand while in the cytoplasm before they can relocate to the nucleus.[35]
Activation
Transcription factors may be activated (or deactivated) through their signal-sensing domain by a number of mechanisms including:
- ligand binding – Not only is ligand binding able to influence where a transcription factor is located within a cell but ligand binding can also affect whether the transcription factor is in an active state and capable of binding DNA or other cofactors (see, for example, nuclear receptors).
- phosphorylation[36][37] – Many transcription factors such as STAT proteins must be phosphorylated before they can bind DNA.
- interaction with other transcription factors (e.g., homo- or hetero-dimerization) or coregulatory proteins
Accessibility of DNA-binding site
In eukaryotes, DNA is organized with the help of histones into compact particles called nucleosomes, where sequences of about 147 DNA base pairs make ~1.65 turns around histone protein octamers. DNA within nucleosomes is inaccessible to many transcription factors. Some transcription factors, so-called pioneering factors are still able to bind their DNA binding sites on the nucleosomal DNA. For most other transcription factors, the nucleosome should be actively unwound by molecular motors such as chromatin remodelers.[38] Alternatively, the nucleosome can be partially unwrapped by thermal fluctuations, allowing temporary access to the transcription factor binding site. In many cases, a transcription factor needs to compete for binding to its DNA binding site with other transcription factors and histones or non-histone chromatin proteins.[39] Pairs of transcription factors and other proteins can play antagonistic roles (activator versus repressor) in the regulation of the same gene.
Availability of other cofactors/transcription factors
Most transcription factors do not work alone. Often, for gene transcription to occur, a number of transcription factors must bind to DNA regulatory sequences. This collection of transcription factors, in turn, recruit intermediary proteins such as cofactors that allow efficient recruitment of the preinitiation complex and RNA polymerase. Thus, for a single transcription factor to initiate transcription, all of these other proteins must also be present, and the transcription factor must be in a state where it can bind to them if necessary. Cofactors are proteins that modulate the effects of transcription factors. Cofactors are interchangeable between specific gene promoters; the protein complex that occupies the promoter DNA and the amino acid sequence of the cofactor determine its spatial conformation. For example, certain steroid receptors can exchange cofactors with NF-κB, which is a switch between inflammation and cellular differentiation; thereby steroids can affect the inflammatory response and function of certain tissues.[40]
Structure

Transcription factors are modular in structure and contain the following domains:[1]
- DNA-binding domain (DBD), which attaches to specific sequences of DNA (enhancer or promoter. Necessary component for all vectors. Used to drive transcription of the vector's transgene promoter sequences) adjacent to regulated genes. DNA sequences that bind transcription factors are often referred to as response elements.
- Trans-activating domain (TAD), which contains binding sites for other proteins such as transcription coregulators. These binding sites are frequently referred to as activation functions (AFs).[41]
- An optional signal sensing domain (SSD) (e.g., a ligand binding domain), which senses external signals and, in response, transmits these signals to the rest of the transcription complex, resulting in up- or down-regulation of gene expression. Also, the DBD and signal-sensing domains may reside on separate proteins that associate within the transcription complex to regulate gene expression.
Trans-activating domain
TAD is domain of the transcription factor that binds other proteins such as transcription coregulators. Proteins containing TADs are Gal4, Gcn4, Oaf1, Leu3, Rtg3, Pho4, Gln3 in yeast and p53, NFAT, NF-κB and VP16 in mammals.[42] Many TADs are as short as 9 amino acids (present in e.g., p53, VP16, MLL, E2A, HSF1, NF-IL6, NFAT1 and NF-κB Gal4, Pdr1, Oaf1, Gcn4, VP16, Pho4, Msn2, Ino2 and P201).
DNA-binding domain

The portion (domain) of the transcription factor that binds DNA is called its DNA-binding domain. Below is a partial list of some of the major families of DNA-binding domains/transcription factors:
Family | InterPro | Pfam | SCOP |
---|---|---|---|
basic helix-loop-helix[43] | InterPro: IPR001092 | Pfam PF00010 | SCOP 47460 |
basic-leucine zipper (bZIP)[44] | InterPro: IPR004827 | Pfam PF00170 | SCOP 57959 |
C-terminal effector domain of the bipartite response regulators | InterPro: IPR001789 | Pfam PF00072 | SCOP 46894 |
AP2/ERF/GCC box | InterPro: IPR001471 | Pfam PF00847 | SCOP 54176 |
helix-turn-helix[45] | |||
homeodomain proteins, which are encoded by homeobox genes, are transcription factors. Homeodomain proteins play critical roles in the regulation of development.[46][47] | InterPro: IPR009057 | Pfam PF00046 | SCOP 46689 |
lambda repressor-like | InterPro: IPR010982 | SCOP 47413 | |
srf-like (serum response factor) | InterPro: IPR002100 | Pfam PF00319 | SCOP 55455 |
paired box[48] | |||
winged helix | InterPro: IPR013196 | Pfam PF08279 | SCOP 46785 |
zinc fingers[49] | |||
* multi-domain Cys2His2 zinc fingers[50] | InterPro: IPR007087 | Pfam PF00096 | SCOP 57667 |
* Zn2/Cys6 | SCOP 57701 | ||
* Zn2/Cys8 nuclear receptor zinc finger | InterPro: IPR001628 | Pfam PF00105 | SCOP 57716 |
Response elements
The DNA sequence that a transcription factor binds to is called a transcription factor-binding site or response element.[51]
Transcription factors interact with their binding sites using a combination of electrostatic (of which hydrogen bonds are a special case) and Van der Waals forces. Due to the nature of these chemical interactions, most transcription factors bind DNA in a sequence specific manner. However, not all bases in the transcription factor-binding site may actually interact with the transcription factor. In addition, some of these interactions may be weaker than others. Thus, transcription factors do not bind just one sequence but are capable of binding a subset of closely related sequences, each with a different strength of interaction.
For example, although the consensus binding site for the TATA-binding protein (TBP) is TATAAAA, the TBP transcription factor can also bind similar sequences such as TATATAT or TATATAA.
Because transcription factors can bind a set of related sequences and these sequences tend to be short, potential transcription factor binding sites can occur by chance if the DNA sequence is long enough. It is unlikely, however, that a transcription factor will bind all compatible sequences in the genome of the cell. Other constraints, such as DNA accessibility in the cell or availability of cofactors may also help dictate where a transcription factor will actually bind. Thus, given the genome sequence it is still difficult to predict where a transcription factor will actually bind in a living cell.
Additional recognition specificity, however, may be obtained through the use of more than one DNA-binding domain (for example tandem DBDs in the same transcription factor or through dimerization of two transcription factors) that bind to two or more adjacent sequences of DNA.
Clinical significance
Transcription factors are of clinical significance for at least two reasons: (1) mutations can be associated with specific diseases, and (2) they can be targets of medications.
Disorders
Due to their important roles in development, intercellular signaling, and cell cycle, some human diseases have been associated with mutations in transcription factors.[52]
Many transcription factors are either tumor suppressors or oncogenes, and, thus, mutations or aberrant regulation of them is associated with cancer. Three groups of transcription factors are known to be important in human cancer: (1) the NF-kappaB and AP-1 families, (2) the STAT family and (3) the steroid receptors.[53]
Below are a few of the more well-studied examples:
Condition | Description | Locus |
---|---|---|
Rett syndrome | Mutations in the MECP2 transcription factor are associated with Rett syndrome, a neurodevelopmental disorder.[54][55] | Xq28 |
Diabetes | A rare form of diabetes called MODY (Maturity onset diabetes of the young) can be caused by mutations in hepatocyte nuclear factors (HNFs)[56] or insulin promoter factor-1 (IPF1/Pdx1).[57] | multiple |
Developmental verbal dyspraxia | Mutations in the FOXP2 transcription factor are associated with developmental verbal dyspraxia, a disease in which individuals are unable to produce the finely coordinated movements required for speech.[58] | 7q31 |
Autoimmune diseases | Mutations in the FOXP3 transcription factor cause a rare form of autoimmune disease called IPEX.[59] | Xp11.23-q13.3 |
Li-Fraumeni syndrome | Caused by mutations in the tumor suppressor p53.[60] | 17p13.1 |
Breast cancer | The STAT family is relevant to breast cancer.[61] | multiple |
Multiple cancers | The HOX family are involved in a variety of cancers.[62] | multiple |
Potential drug targets
Approximately 10% of currently prescribed drugs directly target the nuclear receptor class of transcription factors.[63] Examples include tamoxifen and bicalutamide for the treatment of breast and prostate cancer, respectively, and various types of anti-inflammatory and anabolic steroids.[64] In addition, transcription factors are often indirectly modulated by drugs through signaling cascades. It might be possible to directly target other less-explored transcription factors such as NF-κB with drugs.[65][66][67][68] Transcription factors outside the nuclear receptor family are thought to be more difficult to target with small molecule therapeutics since it is not clear that they are "drugable" but progress has been made on the notch pathway.[69]
Role in evolution
Gene duplications have played a crucial role in the evolution of species. This applies particularly to transcription factors. Once they occur as duplicates, accumulated mutations encoding for one copy can take place without negatively affecting the regulation of downstream targets. However, changes of the DNA binding specificities of the single-copy LEAFY transcription factor, which occurs in most land plants, have recently been elucidated. In that respect, a single-copy transcription factor can undergo a change of specificity through a promiscuous intermediate without losing function. Similar mechanisms have been proposed in the context of all alternative phylogenetic hypotheses, and the role of transcription factors in the evolution of all species.[70][71]
Analysis
There are different technologies available to analyze transcription factors. On the genomic level, DNA-sequencing[72] and database research are commonly used[73] The protein version of the transcription factor is detectable by using specific antibodies. The sample is detected on a western blot. By using electrophoretic mobility shift assay (EMSA),[74] the activation profile of transcription factors can be detected. A multiplex approach for activation profiling is a TF chip system where several different transcription factors can be detected in parallel. This technology is based on DNA microarrays, providing the specific DNA-binding sequence for the transcription factor protein on the array surface.[75]
Classes
As described in more detail below, transcription factors may be classified by their (1) mechanism of action, (2) regulatory function, or (3) sequence homology (and hence structural similarity) in their DNA-binding domains.
Mechanistic
There are two mechanistic classes of transcription factors:
- General transcription factors are involved in the formation of a preinitiation complex. The most common are abbreviated as TFIIA, TFIIB, TFIID, TFIIE, TFIIF, and TFIIH. They are ubiquitous and interact with the core promoter region surrounding the transcription start site(s) of all class II genes.[76]
- Upstream transcription factors are proteins that bind somewhere upstream of the initiation site to stimulate or repress transcription. These are roughly synonymous with specific transcription factors, because they vary considerably depending on what recognition sequences are present in the proximity of the gene.[77]
Examples of specific transcription factors[77] | |||
---|---|---|---|
Factor | Structural type | Recognition sequence | Binds as |
SP1 | Zinc finger | 5'-GGGCGG-3' | Monomer |
AP-1 | Basic zipper | 5'-TGA(G/C)TCA-3' | Dimer |
C/EBP | Basic zipper | 5'-ATTGCGCAAT-3' | Dimer |
Heat shock factor | Basic zipper | 5'-XGAAX-3' | Trimer |
ATF/CREB | Basic zipper | 5'-TGACGTCA-3' | Dimer |
c-Myc | Basic helix-loop-helix | 5'-CACGTG-3' | Dimer |
Oct-1 | Helix-turn-helix | 5'-ATGCAAAT-3' | Monomer |
NF-1 | Novel | 5'-TTGGCXXXXXGCCAA-3' | Dimer |
(G/C) = G or C X = A, T, G or C |
Functional
Transcription factors have been classified according to their regulatory function:[8]
- I. constitutively active – present in all cells at all times – general transcription factors, Sp1, NF1, CCAAT
- II. conditionally active – requires activation
- II.A developmental (cell specific) – expression is tightly controlled, but, once expressed, require no additional activation – GATA, HNF, PIT-1, MyoD, Myf5, Hox, Winged Helix
- II.B signal-dependent – requires external signal for activation
- II.B.1 extracellular ligand (endocrine or paracrine)-dependent – nuclear receptors
- II.B.2 intracellular ligand (autocrine)-dependent - activated by small intracellular molecules – SREBP, p53, orphan nuclear receptors
- II.B.3 cell membrane receptor-dependent – second messenger signaling cascades resulting in the phosphorylation of the transcription factor
Structural
Transcription factors are often classified based on the sequence similarity and hence the tertiary structure of their DNA-binding domains:[78][79][80]
- 1 Superclass: Basic Domains
- 1.1 Class: Leucine zipper factors (bZIP)
- 1.2 Class: Helix-loop-helix factors (bHLH)
- 1.2.1 Family: Ubiquitous (class A) factors
- 1.2.2 Family: Myogenic transcription factors (MyoD)
- 1.2.3 Family: Achaete-Scute
- 1.2.4 Family: Tal/Twist/Atonal/Hen
- 1.3 Class: Helix-loop-helix / leucine zipper factors (bHLH-ZIP)
- 1.4 Class: NF-1
- 1.5 Class: RF-X
- 1.6 Class: bHSH
- 2 Superclass: Zinc-coordinating DNA-binding domains
- 2.1 Class: Cys4 zinc finger of nuclear receptor type
- 2.1.1 Family: Steroid hormone receptors
- 2.1.2 Family: Thyroid hormone receptor-like factors
- 2.2 Class: diverse Cys4 zinc fingers
- 2.2.1 Family: GATA-Factors
- 2.3 Class: Cys2His2 zinc finger domain
- 2.4 Class: Cys6 cysteine-zinc cluster
- 2.5 Class: Zinc fingers of alternating composition
- 2.1 Class: Cys4 zinc finger of nuclear receptor type
- 3 Superclass: Helix-turn-helix
- 3.1 Class: Homeo domain
- 3.1.1 Family: Homeo domain only; includes Ubx
- 3.1.2 Family: POU domain factors; includes Oct
- 3.1.3 Family: Homeo domain with LIM region
- 3.1.4 Family: homeo domain plus zinc finger motifs
- 3.2 Class: Paired box
- 3.2.1 Family: Paired plus homeo domain
- 3.2.2 Family: Paired domain only
- 3.3 Class: Fork head / winged helix
- 3.3.1 Family: Developmental regulators; includes forkhead
- 3.3.2 Family: Tissue-specific regulators
- 3.3.3 Family: Cell-cycle controlling factors
- 3.3.0 Family: Other regulators
- 3.4 Class: Heat Shock Factors
- 3.4.1 Family: HSF
- 3.5 Class: Tryptophan clusters
- 3.5.1 Family: Myb
- 3.5.2 Family: Ets-type
- 3.5.3 Family: Interferon regulatory factors
- 3.6 Class: TEA ( transcriptional enhancer factor) domain
- 3.1 Class: Homeo domain
- 4 Superclass: beta-Scaffold Factors with Minor Groove Contacts
- 4.1 Class: RHR (Rel homology region)
- 4.2 Class: STAT
- 4.2.1 Family: STAT
- 4.3 Class: p53
- 4.3.1 Family: p53
- 4.4 Class: MADS box
- 4.4.1 Family: Regulators of differentiation; includes (Mef2)
- 4.4.2 Family: Responders to external signals, SRF (serum response factor) (SRF)
- 4.4.3 Family: Metabolic regulators (ARG80)
- 4.5 Class: beta-Barrel alpha-helix transcription factors
- 4.6 Class: TATA binding proteins
- 4.6.1 Family: TBP
- 4.7 Class: HMG-box
- 4.8 Class: Heteromeric CCAAT factors
- 4.8.1 Family: Heteromeric CCAAT factors
- 4.9 Class: Grainyhead
- 4.9.1 Family: Grainyhead
- 4.10 Class: Cold-shock domain factors
- 4.10.1 Family: csd
- 4.11 Class: Runt
- 4.11.1 Family: Runt
- 0 Superclass: Other Transcription Factors
See also
- Cdx protein family
- DNA-binding protein
- Inhibitor of DNA-binding protein
- Nuclear receptor, a class of ligand activated transcription factors
- Phylogenetic footprinting
- TRANSFAC database
References
- 1 2 Latchman DS (Dec 1997). "Transcription factors: an overview". The International Journal of Biochemistry & Cell Biology. 29 (12): 1305–12. doi:10.1016/S1357-2725(97)00085-X. PMID 9570129.
- ↑ Karin M (Feb 1990). "Too many transcription factors: positive and negative interactions". The New Biologist. 2 (2): 126–31. PMID 2128034.
- ↑ Roeder RG (Sep 1996). "The role of general initiation factors in transcription by RNA polymerase II". Trends in Biochemical Sciences. 21 (9): 327–35. doi:10.1016/0968-0004(96)10050-5. PMID 8870495.
- ↑ Nikolov DB, Burley SK (Jan 1997). "RNA polymerase II transcription initiation: a structural view". Proceedings of the National Academy of Sciences of the United States of America. 94 (1): 15–22. Bibcode:1997PNAS...94...15N. doi:10.1073/pnas.94.1.15. PMC 33652
. PMID 8990153.
- ↑ Lee TI, Young RA (2000). "Transcription of eukaryotic protein-coding genes". Annual Review of Genetics. 34: 77–137. doi:10.1146/annurev.genet.34.1.77. PMID 11092823.
- ↑ Mitchell PJ, Tjian R (Jul 1989). "Transcriptional regulation in mammalian cells by sequence-specific DNA binding proteins". Science. 245 (4916): 371–8. Bibcode:1989Sci...245..371M. doi:10.1126/science.2667136. PMID 2667136.
- ↑ Ptashne M, Gann A (Apr 1997). "Transcriptional activation by recruitment". Nature. 386 (6625): 569–77. Bibcode:1997Natur.386..569P. doi:10.1038/386569a0. PMID 9121580.
- 1 2 3 Brivanlou AH, Darnell JE (Feb 2002). "Signal transduction and the control of gene expression". Science. 295 (5556): 813–8. Bibcode:2002Sci...295..813B. doi:10.1126/science.1066355. PMID 11823631.
- ↑ van Nimwegen E (Sep 2003). "Scaling laws in the functional content of genomes". Trends in Genetics. 19 (9): 479–84. doi:10.1016/S0168-9525(03)00203-8. PMID 12957540.
- ↑ Babu MM, Luscombe NM, Aravind L, Gerstein M, Teichmann SA (Jun 2004). "Structure and evolution of transcriptional regulatory networks". Current Opinion in Structural Biology. 14 (3): 283–91. doi:10.1016/j.sbi.2004.05.004. PMID 15193307.
- ↑ List Of All Transcription Factors In Human
- ↑ Gill G (2001). "Regulation of the initiation of eukaryotic transcription". Essays in Biochemistry. 37: 33–43. doi:10.1042/bse0370033. PMID 11758455.
- ↑ Narlikar GJ, Fan HY, Kingston RE (Feb 2002). "Cooperation between complexes that regulate chromatin structure and transcription". Cell. 108 (4): 475–87. doi:10.1016/S0092-8674(02)00654-2. PMID 11909519.
- ↑ Xu L, Glass CK, Rosenfeld MG (Apr 1999). "Coactivator and corepressor complexes in nuclear receptor function". Current Opinion in Genetics & Development. 9 (2): 140–7. doi:10.1016/S0959-437X(99)80021-5. PMID 10322133.
- ↑ Robert O. J. Weinzierl (1999). Mechanisms of Gene Expression: Structure, Function and Evolution of the Basal Transcriptional Machinery. World Scientific Publishing Company. ISBN 1-86094-126-5.
- ↑ Reese JC (Apr 2003). "Basal transcription factors". Current Opinion in Genetics & Development. 13 (2): 114–8. doi:10.1016/S0959-437X(03)00013-3. PMID 12672487.
- ↑ Shilatifard A, Conaway RC, Conaway JW (2003). "The RNA polymerase II elongation complex". Annual Review of Biochemistry. 72: 693–715. doi:10.1146/annurev.biochem.72.121801.161551. PMID 12676794.
- ↑ Thomas MC, Chiang CM (2006). "The general transcription machinery and general cofactors". Critical Reviews in Biochemistry and Molecular Biology. 41 (3): 105–78. doi:10.1080/10409230600648736. PMID 16858867.
- ↑ Lobe CG (1992). "Transcription factors and mammalian development". Current Topics in Developmental Biology. Current Topics in Developmental Biology. 27: 351–83. doi:10.1016/S0070-2153(08)60539-6. ISBN 978-0-12-153127-0. PMID 1424766.
- ↑ Lemons D, McGinnis W (Sep 2006). "Genomic evolution of Hox gene clusters". Science. 313 (5795): 1918–22. Bibcode:2006Sci...313.1918L. doi:10.1126/science.1132040. PMID 17008523.
- ↑ Moens CB, Selleri L (Mar 2006). "Hox cofactors in vertebrate development". Developmental Biology. 291 (2): 193–206. doi:10.1016/j.ydbio.2005.10.032. PMID 16515781.
- ↑ Ottolenghi C, Uda M, Crisponi L, Omari S, Cao A, Forabosco A, Schlessinger D (Jan 2007). "Determination and stability of sex". BioEssays. 29 (1): 15–25. doi:10.1002/bies.20515. PMID 17187356.
- ↑ Pawson T (1993). "Signal transduction--a conserved pathway from the membrane to the nucleus". Developmental Genetics. 14 (5): 333–8. doi:10.1002/dvg.1020140502. PMID 8293575.
- ↑ Osborne CK, Schiff R, Fuqua SA, Shou J (Dec 2001). "Estrogen receptor: current understanding of its activation and modulation". Clinical Cancer Research. 7 (12 Suppl): 4338s–4342s; discussion 4411s–4412s. PMID 11916222.
- ↑ Shamovsky I, Nudler E (Mar 2008). "New insights into the mechanism of heat shock response activation". Cellular and Molecular Life Sciences. 65 (6): 855–61. doi:10.1007/s00018-008-7458-y. PMID 18239856.
- ↑ Benizri E, Ginouvès A, Berra E (Apr 2008). "The magic of the hypoxia-signaling cascade". Cellular and Molecular Life Sciences. 65 (7-8): 1133–49. doi:10.1007/s00018-008-7472-0. PMID 18202826.
- ↑ Weber LW, Boll M, Stampfl A (Nov 2004). "Maintaining cholesterol homeostasis: sterol regulatory element-binding proteins". World Journal of Gastroenterology. 10 (21): 3081–7. doi:10.3748/wjg.v10.i21.3081. PMID 15457548.
- ↑ Wheaton K, Atadja P, Riabowol K (1996). "Regulation of transcription factor activity during cellular aging". Biochemistry and Cell Biology = Biochimie Et Biologie Cellulaire. 74 (4): 523–34. doi:10.1139/o96-056. PMID 8960358.
- ↑ Meyyappan M, Atadja PW, Riabowol KT (1996). "Regulation of gene expression and transcription factor binding activity during cellular aging". Biological Signals. 5 (3): 130–8. doi:10.1159/000109183. PMID 8864058.
- ↑ Evan G, Harrington E, Fanidi A, Land H, Amati B, Bennett M (Aug 1994). "Integrated control of cell proliferation and cell death by the c-myc oncogene". Philosophical Transactions of the Royal Society of London. Series B, Biological Sciences. 345 (1313): 269–75. doi:10.1098/rstb.1994.0105. PMID 7846125.
- ↑ Boch J, Bonas U (2010). "Xanthomonas AvrBs3 family-type III effectors: discovery and function". Annual Review of Phytopathology. 48: 419–36. doi:10.1146/annurev-phyto-080508-081936. PMID 19400638.
- ↑ Moscou MJ, Bogdanove AJ (Dec 2009). "A simple cipher governs DNA recognition by TAL effectors". Science. 326 (5959): 1501. Bibcode:2009Sci...326.1501M. doi:10.1126/science.1178817. PMID 19933106.
- ↑ Boch J, Scholze H, Schornack S, Landgraf A, Hahn S, Kay S, Lahaye T, Nickstadt A, Bonas U (Dec 2009). "Breaking the code of DNA binding specificity of TAL-type III effectors". Science. 326 (5959): 1509–12. Bibcode:2009Sci...326.1509B. doi:10.1126/science.1178811. PMID 19933107.
- ↑ Voytas DF, Joung JK (Dec 2009). "Plant science. DNA binding made easy". Science. 326 (5959): 1491–2. Bibcode:2009Sci...326.1491V. doi:10.1126/science.1183604. PMID 20007890.
- 1 2 Whiteside ST, Goodbourn S (Apr 1993). "Signal transduction and nuclear targeting: regulation of transcription factor activity by subcellular localisation". Journal of Cell Science. 104 (4): 949–55. PMID 8314906.
- ↑ Bohmann D (Nov 1990). "Transcription factor phosphorylation: a link between signal transduction and the regulation of gene expression". Cancer Cells. 2 (11): 337–44. PMID 2149275.
- ↑ Weigel NL, Moore NL (Oct 2007). "Steroid receptor phosphorylation: a key modulator of multiple receptor functions". Molecular Endocrinology. 21 (10): 2311–9. doi:10.1210/me.2007-0101. PMID 17536004.
- ↑ Teif VB, Rippe K (Sep 2009). "Predicting nucleosome positions on the DNA: combining intrinsic sequence preferences and remodeler activities". Nucleic Acids Research. 37 (17): 5641–55. doi:10.1093/nar/gkp610. PMC 2761276
. PMID 19625488.
- ↑ Teif VB, Rippe K (Oct 2010). "Statistical-mechanical lattice models for protein-DNA binding in chromatin". Journal of Physics. Condensed Matter. 22 (41): 414105. arXiv:1004.5514
. Bibcode:2010JPCM...22O4105T. doi:10.1088/0953-8984/22/41/414105. PMID 21386588.
- ↑ Copland JA, Sheffield-Moore M, Koldzic-Zivanovic N, Gentry S, Lamprou G, Tzortzatou-Stathopoulou F, Zoumpourlis V, Urban RJ, Vlahopoulos SA (Jun 2009). "Sex steroid receptors in skeletal differentiation and epithelial neoplasia: is tissue-specific intervention possible?". BioEssays. 31 (6): 629–41. doi:10.1002/bies.200800138. PMID 19382224.
- ↑ Wärnmark A, Treuter E, Wright AP, Gustafsson JA (Oct 2003). "Activation functions 1 and 2 of nuclear receptors: molecular strategies for transcriptional activation". Molecular Endocrinology. 17 (10): 1901–9. doi:10.1210/me.2002-0384. PMID 12893880.
- ↑ Piskacek S, Gregor M, Nemethova M, Grabner M, Kovarik P, Piskacek M (Jun 2007). "Nine-amino-acid transactivation domain: establishment and prediction utilities". Genomics. 89 (6): 756–68. doi:10.1016/j.ygeno.2007.02.003. PMID 17467953.
- ↑ Littlewood TD, Evan GI (1995). "Transcription factors 2: helix-loop-helix". Protein Profile. 2 (6): 621–702. PMID 7553065.
- ↑ Vinson C, Myakishev M, Acharya A, Mir AA, Moll JR, Bonovich M (Sep 2002). "Classification of human B-ZIP proteins based on dimerization properties". Molecular and Cellular Biology. 22 (18): 6321–35. doi:10.1128/MCB.22.18.6321-6335.2002. PMC 135624
. PMID 12192032.
- ↑ Wintjens R, Rooman M (Sep 1996). "Structural classification of HTH DNA-binding domains and protein-DNA interaction modes". Journal of Molecular Biology. 262 (2): 294–313. doi:10.1006/jmbi.1996.0514. PMID 8831795.
- ↑ Gehring WJ, Affolter M, Bürglin T (1994). "Homeodomain proteins". Annual Review of Biochemistry. 63: 487–526. doi:10.1146/annurev.bi.63.070194.002415. PMID 7979246.
- ↑ Bürglin TR, Affolter M (Oct 2015). "Homeodomain proteins: an update". Chromosoma. Oct 13. doi:10.1007/s00412-015-0543-8. PMID 26464018.
- ↑ Dahl E, Koseki H, Balling R (Sep 1997). "Pax genes and organogenesis". BioEssays. 19 (9): 755–65. doi:10.1002/bies.950190905. PMID 9297966.
- ↑ Laity JH, Lee BM, Wright PE (Feb 2001). "Zinc finger proteins: new insights into structural and functional diversity". Current Opinion in Structural Biology. 11 (1): 39–46. doi:10.1016/S0959-440X(00)00167-6. PMID 11179890.
- ↑ Wolfe SA, Nekludova L, Pabo CO (2000). "DNA recognition by Cys2His2 zinc finger proteins". Annual Review of Biophysics and Biomolecular Structure. 29: 183–212. doi:10.1146/annurev.biophys.29.1.183. PMID 10940247.
- ↑ Wang JC (Mar 2005). "Finding primary targets of transcriptional regulators". Cell Cycle. 4 (3): 356–8. doi:10.4161/cc.4.3.1521. PMID 15711128.
- ↑ Semenza, Gregg L. (1999). Transcription factors and human disease. Oxford [Oxfordshire]: Oxford University Press. ISBN 0-19-511239-3.
- ↑ Libermann TA, Zerbini LF (Feb 2006). "Targeting transcription factors for cancer gene therapy". Current Gene Therapy. 6 (1): 17–33. doi:10.2174/156652306775515501. PMID 16475943.
- ↑ Moretti P, Zoghbi HY (Jun 2006). "MeCP2 dysfunction in Rett syndrome and related disorders". Current Opinion in Genetics & Development. 16 (3): 276–81. doi:10.1016/j.gde.2006.04.009. PMID 16647848.
- ↑ Chadwick LH, Wade PA (Apr 2007). "MeCP2 in Rett syndrome: transcriptional repressor or chromatin architectural protein?". Current Opinion in Genetics & Development. 17 (2): 121–5. doi:10.1016/j.gde.2007.02.003. PMID 17317146.
- ↑ Maestro MA, Cardalda C, Boj SF, Luco RF, Servitja JM, Ferrer J (2007). "Distinct roles of HNF1beta, HNF1alpha, and HNF4alpha in regulating pancreas development, beta-cell function and growth". Endocrine Development. 12: 33–45. doi:10.1159/0000109603 (inactive 2015-01-01). PMID 17923767.
- ↑ Al-Quobaili F, Montenarh M (Apr 2008). "Pancreatic duodenal homeobox factor-1 and diabetes mellitus type 2 (review)". International Journal of Molecular Medicine. 21 (4): 399–404. doi:10.3892/ijmm.21.4.399. PMID 18360684.
- ↑ Lennon PA, Cooper ML, Peiffer DA, Gunderson KL, Patel A, Peters S, Cheung SW, Bacino CA (Apr 2007). "Deletion of 7q31.1 supports involvement of FOXP2 in language impairment: clinical report and review". American Journal of Medical Genetics Part A. 143A (8): 791–8. doi:10.1002/ajmg.a.31632. PMID 17330859.
- ↑ van der Vliet HJ, Nieuwenhuis EE (2007). "IPEX as a result of mutations in FOXP3". Clinical & Developmental Immunology. 2007: 89017. doi:10.1155/2007/89017. PMC 2248278
. PMID 18317533.
- ↑ Iwakuma T, Lozano G, Flores ER (Jul 2005). "Li-Fraumeni syndrome: a p53 family affair". Cell Cycle. 4 (7): 865–7. doi:10.4161/cc.4.7.1800. PMID 15917654.
- ↑ "Roles and Regulation of Stat Family Transcription Factors in Human Breast Cancer" 2004
- ↑ "Transcription factors as targets and markers in cancer" Workshop 2007
- ↑ Overington JP, Al-Lazikani B, Hopkins AL (Dec 2006). "How many drug targets are there?". Nature Reviews. Drug Discovery. 5 (12): 993–6. doi:10.1038/nrd2199. PMID 17139284.
- ↑ Gronemeyer H, Gustafsson JA, Laudet V (Nov 2004). "Principles for modulation of the nuclear receptor superfamily". Nature Reviews. Drug Discovery. 3 (11): 950–64. doi:10.1038/nrd1551. PMID 15520817.
- ↑ Bustin SA, McKay IA (Jun 1994). "Transcription factors: targets for new designer drugs". British Journal of Biomedical Science. 51 (2): 147–57. PMID 8049612.
- ↑ Butt TR, Karathanasis SK (1995). "Transcription factors as drug targets: opportunities for therapeutic selectivity". Gene Expression. 4 (6): 319–36. PMID 7549464.
- ↑ Papavassiliou AG (Aug 1998). "Transcription-factor-modulating agents: precision and selectivity in drug design". Molecular Medicine Today. 4 (8): 358–66. doi:10.1016/S1357-4310(98)01303-3. PMID 9755455.
- ↑ Ghosh D, Papavassiliou AG (2005). "Transcription factor therapeutics: long-shot or lodestone". Current Medicinal Chemistry. 12 (6): 691–701. doi:10.2174/0929867053202197. PMID 15790306.
- ↑ Moellering RE, Cornejo M, Davis TN, Del Bianco C, Aster JC, Blacklow SC, Kung AL, Gilliland DG, Verdine GL, Bradner JE (Nov 2009). "Direct inhibition of the NOTCH transcription factor complex". Nature. 462 (7270): 182–8. Bibcode:2009Natur.462..182M. doi:10.1038/nature08543. PMC 2951323
. PMID 19907488. Lay summary – The Scientist.
- ↑ Sayou C, Monniaux M, Nanao MH, Moyroud E, Brockington SF, Thévenon E, Chahtane H, Warthmann N, Melkonian M, Zhang Y, Wong GK, Weigel D, Parcy F, Dumas R (2014). "A promiscuous intermediate underlies the evolution of LEAFY DNA binding specificity". Science (New York, N.Y.). 343 (6171): 645–8. doi:10.1126/science.1248229. PMID 24436181.
- ↑ Jin J, He K, Tang X, Li Z, Lv L, Zhao Y, Luo J, Gao G (2015). "An Arabidopsis Transcriptional Regulatory Map Reveals Distinct Functional and Evolutionary Features of Novel Transcription Factors". Molecular Biology and Evolution. 32 (7): 1767–73. doi:10.1093/molbev/msv058. PMC 4476157
. PMID 25750178.
- ↑ EntrezGene database
- ↑ Grau J, Ben-Gal I, Posch S, Grosse I (2006). "VOMBAT: prediction of transcription factor binding sites using variable order Bayesian trees" (PDF). Nucleic Acids Research. 34 (Web Server issue): W529–33. doi:10.1093/nar/gkl212. PMC 1538886
. PMID 16845064.
- ↑ Wenta N, Strauss H, Meyer S, Vinkemeier U (Jul 2008). "Tyrosine phosphorylation regulates the partitioning of STAT1 between different dimer conformations". Proceedings of the National Academy of Sciences of the United States of America. 105 (27): 9238–43. Bibcode:2008PNAS..105.9238W. doi:10.1073/pnas.0802130105. PMC 2453697
. PMID 18591661.
- ↑ Sermeus A, Cosse JP, Crespin M, Mainfroid V, de Longueville F, Ninane N, Raes M, Remacle J, Michiels C (2008). "Hypoxia induces protection against etoposide-induced apoptosis: molecular profiling of changes in gene expression and transcription factor activity". Molecular Cancer. 7: 27. doi:10.1186/1476-4598-7-27. PMC 2330149
. PMID 18366759.
- ↑ Orphanides G, Lagrange T, Reinberg D (Nov 1996). "The general transcription factors of RNA polymerase II". Genes & Development. 10 (21): 2657–83. doi:10.1101/gad.10.21.2657. PMID 8946909.
- 1 2 Walter F. Boron (2003). Medical Physiology: A Cellular And Molecular Approaoch. Elsevier/Saunders. pp. 125–126. ISBN 1-4160-2328-3.
- ↑ Stegmaier P, Kel AE, Wingender E (2004). "Systematic DNA-binding domain classification of transcription factors". Genome Informatics. International Conference on Genome Informatics. 15 (2): 276–86. PMID 15706513. Archived from the original on 19 June 2013.
- ↑ Matys V, Kel-Margoulis OV, Fricke E, Liebich I, Land S, Barre-Dirrie A, Reuter I, Chekmenev D, Krull M, Hornischer K, Voss N, Stegmaier P, Lewicki-Potapov B, Saxel H, Kel AE, Wingender E (Jan 2006). "TRANSFAC and its module TRANSCompel: transcriptional gene regulation in eukaryotes". Nucleic Acids Research. 34 (Database issue): D108–10. doi:10.1093/nar/gkj143. PMC 1347505
. PMID 16381825.
- ↑ "TRANSFAC database". Retrieved 5 August 2007.
External links
- Transcription Factors at the US National Library of Medicine Medical Subject Headings (MeSH)
- Transcription factor database
- Plant Transcription Factor Database and Transcriptional Regulation Data and Analysis Platform