Lanthanum aluminate-strontium titanate interface

The interface between lanthanum aluminate (LaAlO3) and strontium titanate (SrTiO3) is a notable materials interface because it exhibits properties not found in its constituent materials. Individually, LaAlO3 and SrTiO3 are non-magnetic insulators, yet LaAlO3/SrTiO3 interfaces can exhibit electrical conductivity,[1] superconductivity,[2] ferromagnetism,[3] large negative in-plane magnetoresistance,[4] and giant persistent photoconductivity.[5] The study of how these properties emerge at the LaAlO3/SrTiO3 interface is a growing area of research in condensed matter physics.
Emergent properties
Conductivity
Under the right conditions, the LaAlO3/SrTiO3 interface is electrically conductive, like a metal. The angular dependence of Shubnikov-de Haas oscillations indicates that the conductivity is two-dimensional,[6] leading many researchers to refer to it as a two-dimensional electron gas (2DEG). Two-dimensional does not mean that the conductivity has zero thickness, but rather than the electrons are confined to only move in two directions. It is also sometimes called a two-dimensional electron liquid (2DEL) to emphasize the importance of inter-electron interactions.[7]
Conditions necessary for conductivity
Not all LaAlO3/SrTiO3 interfaces are conductive. Typically, conductivity is achieved only when:
- The LaAlO3/SrTiO3 interface is along the 001 crystallographic direction
- The LaAlO3 and SrTiO3 are crystalline and epitaxial
- The SrTiO3 side of the interface is TiO2-terminated (causing the LaAlO3 side of the interface to be LaO-terminated)[1]
- The LaAlO3 layer is at least 4 unit cells thick[8]
Conductivity can also be achieved when the SrTiO3 is doped with oxygen vacancies; however, in that case, the interface is technically LaAlO3/SrTiO3-x instead of LaAlO3/SrTiO3.
Hypotheses for conductivity
The source of conductivity at the LaAlO3/SrTiO3 interface has been debated for years. SrTiO3 is a wide-band gap semiconductor that can be doped n-type in a variety of ways. Clarifying the mechanism behind the conductivity is a major goal of current research. Four leading hypotheses are:
- Polar gating
- Oxygen vacancies
- Intermixing
- Structural distortions
Polar gating
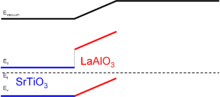
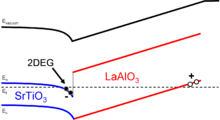
Polar gating was the first mechanism used to explain the conductivity at LaAlO3/SrTiO3 interfaces.[1] It postulates that the LaAlO3, which is polar in the 001 direction (with alternating sheets of positive and negative charge), acts as an electrostatic gate on the semiconducting SrTiO3.[1] When the LaAlO3 layer grows thicker than three unit cells, its valence band energy rises above the Fermi level, causing holes (or positively charged oxygen vacancies[9] ) to form on the outer surface of the LaAlO3. The positive charge on the surface of the LaAlO3 attracts negative charge to nearby available states. In the case of the LaAlO3/SrTiO3 interface, this means electrons accumulate in the surface of the SrTiO3, in the Ti d bands.
The strengths of the polar gating hypothesis are that it explains why conductivity requires a critical thickness of four unit cells of LaAlO3 and that it explains why conductivity requires the SrTiO3 to be TiO2-terminated. The polar gating hypothesis also explains why alloying the LaAlO3 increases the critical thickness for conductivity.[10]
One weakness of the hypothesis is that it predicts that the LaAlO3 films should exhibit a built-in electric field; so far, x-ray photoemission experiments[11][12][13][14] and other experiments[15][16][17] have shown little to no built-in field in the LaAlO3 films. The polar gating hypothesis also cannot explain why Ti3+ is detected when the LaAlO3 films are thinner than the critical thickness for conductivity.[12]
The polar gating hypothesis is sometimes called the polar catastrophe hypothesis,[18] alluding to the counterfactual scenario where electrons don't accumulate at the interface and instead voltage in the LaAlO3 builds up forever. The hypothesis has also been called the electronic reconstruction hypothesis,[18] highlighting the fact that electrons, not ions, move to compensate the building voltage.
Oxygen vacancies
Another hypothesis is that the conductivity comes from free electrons left by oxygen vacancies in the SrTiO3.[19] SrTiO3 is known to be easily doped by oxygen vacancies, so this was initially considered a promising hypothesis. However, electron energy loss spectroscopy measurements have bounded the density of oxygen vacancies well below the density necessary to supply the measured free electron densities.[20] Another proposed possibility is that oxygen vacancies in the surface of the LaAlO3 are remotely doping the SrTiO3.[12] Under generic growth conditions, multiple mechanisms can coexist. A systematic study [21] across a wide growth parameter space demonstrated different roles played by oxygen vacancy formation and the polar gating at different interfaces. An obvious difference between oxygen vacancies and polar gating in creating the interface conductivity is that the carriers from oxygen vacancies are thermally activated as the donor level of oxygen vacancies is usually separated from the SrTiO3 conduction band, consequently exhibiting the carrier freeze-out effect[22] at low temperatures; in contrast, the carriers originating from the polar gating are transferred into the SrTiO3 conduction band (Ti 3d orbitals) and are therefore degenerate.[21]
Intermixing
Lanthanum is a known dopant in SrTiO3,[23] so it has been suggested that La from the LaAlO3 mixes into the SrTiO3 and dopes it n-type. Multiple studies have shown that intermixing takes place at the interface;[24] however, it is not clear whether there is enough intermixing to provide all of the free carriers. For example, a flipped interface between a SrTiO3 film and a LaAlO3 substrate is insulating.[25]
Structural distortions
A fourth hypothesis is that the LaAlO3 crystal structure undergoes octahedral rotations in response to the strain from the SrTiO3. These octahedral rotations in the LaAlO3 induce octahedral rotations in the SrTiO3, increasing the Ti d-band width enough so that electrons are no longer localized.[26]
Superconductivity
Superconductivity was first observed in LaAlO3/SrTiO3 interfaces in 2007, with a critical temperature of ~200 mK.[27] Like the conductivity, the superconductivity appears to be two-dimensional.[2]
Ferromagnetism
Hints of ferromagnetism in LaAlO3/SrTiO3 were first seen in 2007, when Dutch researchers observed hysteresis in the magnetoresistance of LaAlO3/SrTiO3.[28] Follow up measurements with torque magnetometry indicated that the magnetism in LaAlO3/SrTiO3 persisted all the way to room temperature.[29] In 2011, researchers at Stanford University used a scanning SQUID to directly image the ferromagnetism, and found that it occurred in heterogeneous patches.[3] Like the conductivity in LaAlO3/SrTiO3, the magnetism only appeared when the LaAlO3 films were thicker than a few unit cells.[30] However, unlike conductivity, magnetism was seen at SrO-terminated surfaces as well as TiO2-terminated surfaces.[30]
The discovery of ferromagnetism in a materials system that also superconducts spurred a flurry of research and debate, because ferromagnetism and superconductivity almost never coexist together.[3] Ferromagnetism requires electron spins to align, while superconductivity typically requires electron spins to anti-align.
Magnetoresistance
Magnetoresistance measurements are a major experimental tool used to understand the electronic properties of materials. The magnetoresistance of LaAlO3/SrTiO3 interfaces has been used to reveal the 2D nature of conduction, carrier concentrations (through the hall effect), electron mobilities, and more.[6]
Field applied out-of-plane
At low magnetic field, the magnetoresistance of LaAlO3/SrTiO3 is parabolic versus field, as expected for an ordinary metal.[31] However, at higher fields, the magnetoresistance appears to becomes linear versus field.[31] Linear magnetoresistance can have many causes, but so far there is no scientific consensus on the cause of linear magnetoresistance in LaAlO3/SrTiO3 interfaces.[31] Linear magnetoresistance has also been measured in pure SrTiO3 crystals,[32] so it may be unrelated to the emergent properties of the interface.
Field applied in-plane
At low temperature (T < 30 K), the LaAlO3/SrTiO3 interface exhibits negative in-plane magnetoresistance,[31] sometimes as large as -90%.[4] The large negative in-plane magnetoresistance has been ascribed to the interface's enhanced spin-orbit interaction.[4][33]
Electron gas distribution at the LaAlO3/SrTiO3 interface
Experimentally, the charge density profile of the electron gas at the LaAlO3/SrTiO3 interface has a strongly asymmetric shape with a rapid initial decay over the first 2 nm and a pronounced tail that extends to about 11 nm.[34][35] A wide variety of theoretical calculations support this result. Importantly, to get electron distribution one have to take into account field-dependent dielectric constant of SrTiO3.[36][37][38]
Comparison to other 2D electron gases
The 2D electron gas that arises at the LaAlO3/SrTiO3 interface is notable for two main reasons. First, it has very high carrier concentration, on the order of 1013 cm−2. Second, if the polar gating hypothesis is true, the 2D electron gas has the potential to be totally free of disorder, unlike other 2D electron gases that require doping or gating to form. However, so far researchers have been unable to synthesize interfaces that realize the promise of low disorder.
Synthesis methods
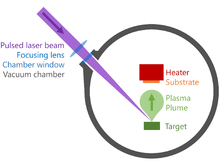
Most LaAlO3/SrTiO3 interfaces are synthesized using pulsed laser deposition. A high-power laser ablates a LaAlO3 target, and the plume of ejected material is deposited onto a heated SrTiO3 substrate. Typical conditions used are:
- Laser wavelength of 248 nm
- Laser fluence of 0.5 J/cm2 to 2 J/cm2[39]
- Substrate temperature of 600 °C to 850 °C[28]
- Background oxygen pressure of 10−5 Torr to 10−3 Torr[28]
Some LaAlO3/SrTiO3 interfaces have also been synthesized by molecular beam epitaxy, sputtering, and atomic layer deposition.[40]
Similar interfaces
To better understand in the LaAlO3/SrTiO3 interface, researchers have synthesized a number of analogous interfaces between other polar perovskite films and SrTiO3. Some of these analogues have properties similar to LaAlO3/SrTiO3, but some do not.
Conductive interfaces
- GdTiO3/SrTiO3[41]
- LaTiO3/SrTiO3[42]
- LaVO3/SrTiO3[42]
- LaGaO3/SrTiO3[43]
- PrAlO3/SrTiO3[44]
- NdAlO3/SrTiO3[44]
- NdGaO3/SrTiO3[44]
- GdAlO3/SrTiO3[45]
- Nd0.35Sr0.65MnO3/SrTiO3[46]
- Al2O3/SrTiO3[47]
- amorphous-YAlO3/SrTiO3[40]
- La0.5Al0.5Sr0.5Ti0.5O3/SrTiO3[10]
- DyScO3/SrTiO3[48]
- KTaO3/SrTiO3[49]
- CaZrO3/SrTiO3[50]
Insulating interfaces
- LaCrO3/SrTiO3[51]
- LaMnO3/SrTiO3[43]
- La2O3/SrTiO3[40]
- Y2O3/SrTiO3[40]
- LaYO3/SrTiO3[40]
- EuAlO3/SrTiO3[45]
- BiMnO3/SrTiO3[52]
Applications
As of 2015, there are no commercial applications of the LaAlO3/SrTiO3 interface. However, speculative applications have been suggested, including field-effect devices, sensors, photodetectors, and thermoelectrics;[53] related LaVO3/SrTiO3 is a functional solar cell[54] albeit hitherto with a low efficiency. [55]
References
- 1 2 3 4 Ohtomo, A.; Hwang (29 Jan 2004). "A high-mobility electron gas at the LaAlO3/SrTiO3 heterointerface". Nature. 427 (6973): 423–426. Bibcode:2004Natur.427..423O. doi:10.1038/nature02308.
- 1 2 Gariglio, S.; Reyren, N.; Caviglia, A. D.; Triscone, J.-M. (31 March 2009). "Superconductivity at the LaAlO3/SrTiO3 interface". Journal of Physics: Condensed Matter. 21 (16): 164213. Bibcode:2009JPCM...21p4213G. doi:10.1088/0953-8984/21/16/164213.
- 1 2 3 Bert, Julie A.; Kalisky, Bell; Kim, Hikita; Hwang, Moler (4 September 2011). "Direct imaging of the coexistence of ferromagnetism and superconductivity at the LaAlO3/SrTiO3 interface". Nature Physics. 7 (10): 767–771. arXiv:1108.3150
. Bibcode:2011NatPh...7..767B. doi:10.1038/nphys2079.
- 1 2 3 Ben Shalom, M.; Sachs, Rakhmilevitch; Palevski, Dagan (26 March 2010). "Tuning Spin-Orbit Coupling and Superconductivity at the SrTiO3/LaAlO3 Interface: A Magnetotransport Study". Physical Review Letters. 104 (12). arXiv:1001.0781
. Bibcode:2010PhRvL.104l6802B. doi:10.1103/PhysRevLett.104.126802.
- ↑ Tebano, Antonello; E Fabbri; D Pergolesi; G Balestrino; E Traversa (19 January 2012). "Room-Temperature Giant Persistent Photoconductivity in SrTiO3/LaAlO3 Heterostructures". ACS Nano. 6 (2): 1278–1283. doi:10.1021/nn203991q.
- 1 2 Caviglia, A. D.; Gariglio, Cancellieri; Sacepe, Fete; Reyren, Gabay; Morpurgo, Triscone (1 December 2010). "Two-Dimensional Quantum Oscillations of the Conductance at LaAlO3/SrTiO3 Interfaces". Physical Review Letters. 105 (23). arXiv:1007.4941
. Bibcode:2010PhRvL.105w6802C. doi:10.1103/PhysRevLett.105.236802.
- ↑ Breitschaft, M; V. Tinkl; N. Pavlenko; S. Paetel; C. Richter; J. R. Kirtley; Y. C. Liao; G. Hammerl; V. Eyert; T. Kopp; J. Mannhart (30 April 2010). "Two-dimensional electron liquid state at LaAlO3-SrTiO3 interfaces". Physical Review B. 81 (15). arXiv:0907.1176
. Bibcode:2010PhRvB..81o3414B. doi:10.1103/PhysRevB.81.153414.
- ↑ Thiel, S.; Hammerl, Schmehl; Schneider, Mannhart (29 September 2006). "Tunable Quasi-Two-Dimensional Electron Gases in Oxide Heterostructures". Science. 313 (5795): 1942–1945. Bibcode:2006Sci...313.1942T. doi:10.1126/science.1131091.
- ↑ Robertson, J.; S. J. Clark (28 Feb 2011). "Limits to doping in oxides". Physical Review B. 83 (7). Bibcode:2011PhRvB..83g5205R. doi:10.1103/PhysRevB.83.075205.
- 1 2 Reinle-Schmitt, M.L.; Cancellieri, Li; Fontaine, Medarde; Pomjakushina, Scheider; Gariglio, Ghosez; Triscone, Willmott (3 July 2012). "Tunable conductivity threshold at polar oxide interfaces". Nature Communications. 3: 932. Bibcode:2012NatCo...3E.932R. doi:10.1038/ncomms1936.
- ↑ Berner, G.; A. Müller; F. Pfaff; J. Walde; C. Richter; J. Mannhart; S. Thiess; A. Gloskovskii; W. Drube; M. Sing; R. Claessen (6 September 2013). "Band alignment in LaAlO3/SrTiO3 oxide heterostructures inferred from hard x-ray photoelectron spectroscopy". Physical Review B. 88 (11). Bibcode:2013PhRvB..88k5111B. doi:10.1103/PhysRevB.88.115111.
- 1 2 3 Slooten, E.; Zhong, Molegraaf, Eerkes, de Jong, Massee, van Heumen, Kruize, Wenderich, Kleibeuker, Gorgoi, Hilgenkamp, Brinkman, Huijben, Rijnders, Blank, Koster, Kelly, Golden (25 February 2013). "Hard x-ray photoemission and density functional theory study of the internal electric field in SrTiO3/LaAlO3 oxide heterostructures". Physical Review B. 87 (8). arXiv:1301.2179
. Bibcode:2013PhRvB..87h5128S. doi:10.1103/PhysRevB.87.085128. Cite uses deprecated parameter
|coauthors=
(help) - ↑ Drera, G.; G. Salvinelli; A. Brinkman; M. Huijben; G. Koster; H. Hilgenkamp; G. Rijnders; D. Visentin; L. Sangaletti (25 February 2013). "Band offsets and density of Ti3+ states probed by x-ray photoemission on LaAlO3/SrTiO3 heterointerfaces and their LaAlO3 and SrTiO3 bulk precursors". Physical Review B. 87 (7). arXiv:1211.5519
. Bibcode:2013PhRvB..87g5435D. doi:10.1103/PhysRevB.87.075435.
- ↑ Segal, Y.; J. H. Ngai; J. W. Reiner; F. J. Walker; C. H. Ahn (23 December 2009). "X-ray photoemission studies of the metal-insulator transition in LaAlO3/SrTiO3 structures grown by molecular beam epitaxy". Physical Review B. 80 (24). Bibcode:2009PhRvB..80x1107S. doi:10.1103/PhysRevB.80.241107.
- ↑ Huang, Bo-Chao; Ya-Ping Chiu, Po-Cheng Huang, Wen-Ching Wang, Vu Thanh Tra, Jan-Chi Yang, Qing He, Jiunn-Yuan Lin, Chia-Seng Chang, and Ying-Hao Chu (12 December 2012). "Mapping Band Alignment across Complex Oxide Heterointerfaces". Physical Review Letters. 109 (24). Bibcode:2012PhRvL.109x6807H. doi:10.1103/PhysRevLett.109.246807. Cite uses deprecated parameter
|coauthors=
(help) - ↑ Cancellieri, C.; D. Fontaine, S. Gariglio, N. Reyren, A. D. Caviglia, A. Fête, S. J. Leake, S. A. Pauli, P. R. Willmott, M. Stengel, Ph Ghosez, and J.-M. Triscone (28 July 2011). "Electrostriction at the LaAlO3/SrTiO3 Interface". Physical Review Letters. 107 (5). Bibcode:2011PhRvL.107e6102C. doi:10.1103/PhysRevLett.107.056102. Cite uses deprecated parameter
|coauthors=
(help) - ↑ Singh-Bhalla, Guneeta; Christopher Bell; Jayakanth Ravichandran; Wolter Siemons; Yasuyuki Hikita; Sayeef Salahuddin; Arthur F. Hebard; Harold Y. Hwang; Ramamoorthy Ramesh (24 October 2010). "Built-in and induced polarization across LaAlO3/SrTiO3 heterojunctions". Nature Physics. 7 (1): 80–86. arXiv:1005.4257
. Bibcode:2011NatPh...7...80S. doi:10.1038/nphys1814.
- 1 2 Savoia, A; D. Paparo, P. Perna, Z. Ristic, M. Salluzzo, F. Miletto Granozio, U. Scotti di Uccio, C. Richter, S. Thiel, J. Mannhart, L. Marrucci (4 September 2009). "Polar catastrophe and electronic reconstructions at the LaAlO3/SrTiO3 interface: Evidence from optical second harmonic generation". Physical Review B. 80 (7): 075110. arXiv:0901.3331
. Bibcode:2009PhRvB..80g5110S. doi:10.1103/PhysRevB.80.075110. Cite uses deprecated parameter
|coauthors=
(help) - ↑ Kalabukhov, Alexey; Robert Gunnarsson; Johan Börjesson; Eva Olsson; Tord Claeson; Dag Winkler (19 March 2007). "Effect of oxygen vacancies in the SrTiO3 substrate on the electrical properties of the LaAlO3∕SrTiO3 interface". Physical Review B. 75 (12). arXiv:cond-mat/0603501
. Bibcode:2007PhRvB..75l1404K. doi:10.1103/PhysRevB.75.121404.
- ↑ Cantoni; Gazquez, Granozio; Oxley, Varela; Lupini, Pennycook; Aruta, Uccio; Perna, Maccariello (19 June 2009). "Electron Transfer and Ionic Displacements at the Origin of the 2D Electron Gas at the LAO/STO Interface: Direct Measurements with Atomic-Column Spatial Resolution". Advanced Materials. 24 (29): 3952. doi:10.1002/adma.201200667.
- 1 2 Z. Q. Liu; C. J. Li, W. M. Lu, Z. Huang, S. W. Zeng, X. P. Qiu, L. S. Huang, A. Annadi, J. S. Chen, J. M. D. Coey, T. Venkatesan, Ariando (30 May 2013). "Origin of the two-dimensional electron gas at LaAlO3/SrTiO3 interfaces - The role of oxygen vacancies and electronic reconstruction". Physical Review X. 3 (2): 021010. arXiv:1305.5016
. Bibcode:2013PhRvX...3b1010L. doi:10.1103/PhysRevX.3.021010. Cite uses deprecated parameter
|coauthors=
(help) - ↑ Z. Q. Liu; D. P. Leusink, X. Wang, M. M. Lu, K. Gopinadhan, A. Annadi, Y. L. Zhao, X. H. Huang, S. W. Zeng, Z. Huang, A. Srivastava, S. Dhar, T. Venkatesan, Ariando (28 September 2011). "Metal-insulator transition in SrTiO3-x thin films induced by frozen-out carriers". Physical Review Letters. 107 (14): 146802. arXiv:1102.5595
. Bibcode:2011PhRvL.107n6802L. doi:10.1103/PhysRevLett.107.146802. Cite uses deprecated parameter
|coauthors=
(help) - ↑ Frederikse, H.P.R.; W.R. Hosler (September 1967). "Hall Mobility in SrTiO3". Phys. Rev. 161 (3): 822–827. Bibcode:1967PhRv..161..822F. doi:10.1103/PhysRev.161.822.
- ↑ Qiao, L; Droubay, Shutthanandan; Zhu, Chambers (16 July 2010). "Thermodynamic instability at the stoichiometric LaAlO3/SrTiO3(001) interface" (PDF). Journal of Physics: Condensed Matter. 22 (31): 312201. Bibcode:2010JPCM...22E2201Q. doi:10.1088/0953-8984/22/31/312201.
- ↑ Z. Q. Liu; Z. Huang, W. M. Lu, K. Gopinadhan, X. Wang, A. Annadi, T. Venkatesan, Ariando (14 February 2012). "Atomically flat interface between a single-terminated LaAlO3 substrate and SrTiO3 thin film is insulating". AIP Advances. 2 (1): 012147. arXiv:1205.1305
. Bibcode:2012AIPA....2a2147L. doi:10.1063/1.3688772. Cite uses deprecated parameter
|coauthors=
(help) - ↑ Schoofs, Frank; Carpenter, Vickers, Egilmez, Fix, Kleibeuker, MacManus-Driscoll, Blamire (8 April 2013). "Carrier density modulation by structural distortions at modified LaAlO3/SrTiO3 interfaces". Journal of Physics: Condensed Matter. 25 (17): 175005. Bibcode:2013JPCM...25q5005S. doi:10.1088/0953-8984/25/17/175005. Cite uses deprecated parameter
|coauthors=
(help) - ↑ Reyren, N.; S. Thiel, A. D. Caviglia, L. Fitting Kourkoutis, G. Hammerl, C. Richter, C. W. Schneider, T. Kopp, A.-S. Rüetschi, D. Jaccard, M. Gabay, D. A. Muller, J.-M. Triscone, J. Mannhart (2 Aug 2007). "Superconducting Interfaces Between Insulating Oxides". Science. 317 (5842): 1196–1199. Bibcode:2007Sci...317.1196R. doi:10.1126/science.1146006. Cite uses deprecated parameter
|coauthors=
(help) - 1 2 3 Brinkman, A.; Huijben, van Zalk, Huijben, Zeitler, Maan, van der Wiel, Rijnders, Blank, Hilgenkamp (3 June 2007). "Magnetic effects at the interface between non-magnetic oxides". Nature Materials. 6 (7): 493–496. arXiv:cond-mat/0703028
. Bibcode:2007NatMa...6..493B. doi:10.1038/nmat1931. Cite uses deprecated parameter
|coauthors=
(help) - ↑ Ariando; X. Wang, G. Baskaran, Z. Q. Liu, J. Huijben, J. B. Yi, A. Annadi, A. Roy Barman, A. Rusydi, S. Dhar, Y. P. Feng, J. Ding, H. Hilgenkamp & T. Venkatesan (8 February 2011). "Electronic phase separation at the LaAlO3/SrTiO3 interface". Nature Communications. 2: 188. Bibcode:2011NatCo...2E.188A. doi:10.1038/ncomms1192. Cite uses deprecated parameter
|coauthors=
(help); horizontal tab character in|coauthors=
at position 9 (help) - 1 2 Kalisky, Beena; Julie A. Bert; Brannon B. Klopfer; Christopher Bell; Hiroki K. Sato; Masayuki Hosoda; Yasuyuki Hikita; Harold Y. Hwang; Kathryn A. Moler (5 January 2012). "Critical thickness for ferromagnetism in LaAlO3/SrTiO3 heterostructures". 3: 922. arXiv:1201.1063
. Bibcode:2012NatCo...3E.922K. doi:10.1038/ncomms1931.
- 1 2 3 4 Wang, X.; Lu, Annadi, Liu, Gopinadhan, Dhar, Venkatesan, Ariando (8 August 2011). "Magnetoresistance of two-dimensional and three-dimensional electron gas in LaAlO3/SrTiO3 heterostructures: Influence of magnetic ordering, interface scattering, and dimensionality". Physical Review B. 84 (7). arXiv:1110.5290
. Bibcode:2011PhRvB..84g5312W. doi:10.1103/PhysRevB.84.075312. Cite uses deprecated parameter
|coauthors=
(help) - ↑ Z. Q. Liu, Z. Q.; W. M. Lu, X. Wang, Z. Huang, A. Annadi, S. W. Zeng, T. Venkatesan, Ariando (6 April 2012). "Magnetic-field induced resistivity minimum with in-plane linear magnetoresistance of the Fermi liquid in SrTiO3-x single crystals". Physical Review B. 85 (15). arXiv:1204.1901
. Bibcode:2012PhRvB..85o5114L. doi:10.1103/PhysRevB.85.155114. Cite uses deprecated parameter
|coauthors=
(help) - ↑ Flekser, E.; Ben Shalom, Kim, Bell, Hikita, Hwang, Dagan (11 September 2012). "Magnetotransport effects in polar versus non-polar SrTiO3 based heterostructures". Physical Review B. 86 (12). arXiv:1207.6057
. Bibcode:2012PhRvB..86l1104F. doi:10.1103/PhysRevB.86.121104. Cite uses deprecated parameter
|coauthors=
(help) - ↑ Dubroka, A.; M. Rössle, K. W. Kim, V. K. Malik, L. Schultz, S. Thiel, C. W. Schneider, J. Mannhart, G. Herranz, O. Copie, M. Bibes, A. Barthélémy, and C. Bernhard (2010). "Dynamical Response and Confinement of the Electrons at the LaAlO3/SrTiO3 Interface". Phys. Rev. Lett. 104. arXiv:0910.0741
. Bibcode:2010PhRvL.104o6807D. doi:10.1103/PhysRevLett.104.156807. Cite uses deprecated parameter
|coauthors=
(help) - ↑ Yamada, Y.; Hiroki K. Sato; Yasuyuki Hikita; Harold Y. Hwang; Yoshihiko Kanemitsu (2014). "Spatial density profile of electrons near the LaAlO3/SrTiO3heterointerface revealed by time-resolved photoluminescence spectroscopy". Appl. Phys. Lett. 104. Bibcode:2014ApPhL.104o1907Y. doi:10.1063/1.4872171.
- ↑ Park, Se Young; Andrew J. Millis (2013). "Charge density distribution and optical response of the LaAlO3/SrTiO3 interface". Phys. Rev. B. 87. arXiv:1302.7290
. Bibcode:2013PhRvB..87t5145P. doi:10.1103/PhysRevB.87.205145.
- ↑ Khalsa, G.; A. H. MacDonald (2012). "Theory of the SrTiO3 surface state two-dimensional electron gas". Phys. Rev. B. 86. arXiv:1205.4362
. Bibcode:2012PhRvB..86l5121K. doi:10.1103/PhysRevB.86.125121.
- ↑ Reich, K.V.; M. Schecter; B. I. Shklovskii (2015). "Accumulation, inversion, and depletion layers in SrTiO3". Phys. Rev. B. 91. arXiv:1412.6024
. Bibcode:2015PhRvB..91k5303R. doi:10.1103/PhysRevB.91.115303.
- ↑ Sato, H. K.; Bell, Hikita, Hwang (25 June 2013). "Stoichiometry control of the electronic properties of the LaAlO3/SrTiO3 heterointerface". Applied Physics Letters. 102 (25): 251602. arXiv:1304.7830
. Bibcode:2013ApPhL.102y1602S. doi:10.1063/1.4812353. Cite uses deprecated parameter
|coauthors=
(help) - 1 2 3 4 5 Lee, Sang Woon; Yiqun Liu; Jaeyeong Heo; Roy G. Gordon (21 August 2012). "Creation and Control of Two-Dimensional Electron Gas Using Al-Based Amorphous Oxides/SrTiO3 Heterostructures Grown by Atomic Layer Deposition". Nano Letters. 12 (9): 4775–4783. Bibcode:2012NanoL..12.4775L. doi:10.1021/nl302214x.
- ↑ Moetakef, Pouya; Cain, Ouellette, Zhang, Klenov, Janotti, Van de Walle, Rajan, Allen, Stemmer (9 December 2011). "Electrostatic carrier doping of GdTiO3/SrTiO3 interfaces". Applied Physics Letters. 99 (23): 232116. arXiv:1111.4684
. Bibcode:2011ApPhL..99w2116M. doi:10.1063/1.3669402. Cite uses deprecated parameter
|coauthors=
(help) - 1 2 He, C.; Sanders, Gray, Wong, Mehta, Suzuki (1 August 2012). "Metal-insulator transitions in epitaxial LaVO3 and LaTiO3 films". Physical Review B. 86 (8). Bibcode:2012PhRvB..86h1401H. doi:10.1103/PhysRevB.86.081401. Cite uses deprecated parameter
|coauthors=
(help) - 1 2 Perna, P.; Maccariello, Radovic, Scott di Uccio, Pallecchi, Codda, Marre, Cantoni, Gazquez, Varela, Pennycook, Granozio (14 October 2010). "Conducting interfaces between band insulating oxides: The LaGaO3/SrTiO3 heterostructure". Applied Physics Letters. 97 (15): 152111. arXiv:1001.3956
. Bibcode:2010ApPhL..97o2111P. doi:10.1063/1.3496440. Cite uses deprecated parameter
|coauthors=
(help) - 1 2 3 Annadi, A.; Putra, Liu; Wang, Gopinadhan; Huang, Dhar; Vekatesan, Ariando (27 August 2012). "Electronic correlation and strain effects at the interfaces between polar and nonpolar complex oxides". Physical Review B. 86 (8). arXiv:1208.0410
. Bibcode:2012PhRvB..86h5450A. doi:10.1103/PhysRevB.86.085450.
- 1 2 Monti, Mark. "The effect of epitaxial strain and R3+ magnetism on the interfaces between polar perovskites and SrTiO3" (PDF). PhD Thesis. University of Texas at Austin. Retrieved 2 August 2013.
- ↑ Chang, C.-P.; J. G. Lin, H. T. Jeng, S.-L. Cheng, W. F. Pong, Y. C. Shao, Y. Y. Chin, H.-J. Lin, C. W. Chen, J.-R. Yang, C. H. Chen, and M.-W. Chu (19 February 2013). "Atomic-scale observation of a graded polar discontinuity and a localized two-dimensional electron density at an insulating oxide interface". Physical Review B. 87 (7). Bibcode:2013PhRvB..87g5129C. doi:10.1103/PhysRevB.87.075129. Cite uses deprecated parameter
|coauthors=
(help) - ↑ Chen, Y. Z.; Bovet, Trier; Christensen, Qu; Andersen, Kasama; Zhang, Giraud; Dufouleur, Jespersen; Sun, SMith; Nygard, Lu; Buchner, Shen; Linderoth, Pryds (22 January 2013). "A high-mobility two-dimensional electron gas at the spinel/perovskite interface of γ-Al2O3/SrTiO3". Nature Communications. 4: 1371. arXiv:1304.0336
. Bibcode:2013NatCo...4E1371C. doi:10.1038/ncomms2394.
- ↑ Li, D.F.; Yan Wang; J.Y. Dai (24 March 2011). "Tunable electronic transport properties of DyScO3/SrTiO3 polar heterointerface". Applied Physics Letters. 98 (12): 122108. Bibcode:2011ApPhL..98l2108L. doi:10.1063/1.3570694.
- ↑ Kalabukhov, A.; R. Gunnarsson; T. Claeson; D. Winkler (9 April 2007). "Electrical transport properties of polar heterointerface between KTaO3 and SrTiO3". arXiv:0704.1050
.
- ↑ Chen, Yunzhong; Felix Trier, Takeshi Kasama, Dennis V. Christensen, Nicolas Bovet, Zoltan I. Balogh, Han Li, Karl Tor Sune Thydén, Wei Zhang, Sadegh Yazdi, Poul Norby, Nini Pryds, and Søren Linderoth (18 Feb 2015). "Creation of High Mobility Two-Dimensional Electron Gases via Strain Induced Polarization at an Otherwise Nonpolar Complex Oxide Interface". Nano Letters. doi:10.1021/nl504622w. Cite uses deprecated parameter
|coauthors=
(help) - ↑ Chambers, S. A.; Qiao, Droubay, Kaspar, Arey, Sushko (7 November 2011). "Band Alignment, Built-In Potential, and the Absence of Conductivity at the LaCrO3/SrTiO3(001) Heterojunction". Physical Review Letters. 107 (20). Bibcode:2011PhRvL.107t6802C. doi:10.1103/PhysRevLett.107.206802. Cite uses deprecated parameter
|coauthors=
(help) - ↑ Salluzzo, M.; S. Gariglio, D. Stornaiuolo, V. Sessi, S. Rusponi, C. Piamonteze, G. M. De Luca, M. Minola, D. Marré, A. Gadaleta, H. Brune, F. Nolting, N. B. Brookes, and G. Ghiringhelli, (22 August 2013). "Origin of Interface Magnetism in BiMnO3/SrTiO3 and LaAlO3/SrTiO3 Heterostructures". Physical Review Letters. 111 (8). arXiv:1305.2226
. Bibcode:2013PhRvL.111h7204S. doi:10.1103/PhysRevLett.111.087204. Cite uses deprecated parameter
|coauthors=
(help) - ↑ Bogorin, Daniela F.; Patrick Irvin; Cheng Cen; Jeremy Levy (24 November 2010). "LaAlO3/SrTiO3-Based Device Concepts". arXiv:1011.5290
.
- ↑ Elias Assmann; Peter Blaha; Robert Laskowski; Karsten Held; Satoshi Okamoto; Giorgio Sangiovanni (2013). "Oxide Heterostructures for Efficient Solar Cells". Phys. Rev. Lett. 110: 078701. arXiv:1301.1314
. Bibcode:2013PhRvL.110g8701A. doi:10.1103/PhysRevLett.110.078701.
- ↑ Lingfei Wang; Yongfeng Li; Ashok Bera; Chun Ma; Feng Jin; Kaidi Yuan; Wanjian Yin; Adrian David; Wei Chen; Wenbin Wu; Wilfrid Prellier; Suhuai Wei; Tom Wu (2015). "Device Performance of the Mott Insulator LaVO3 as a Photovoltaic Material". Physical Review Applied. 3: 064015. Bibcode:2015PhRvP...3f4015W. doi:10.1103/PhysRevApplied.3.064015.